- Organization
-
IAU Double Star Center
- WDS Description
-
WDS Catalog
- WDS Catalog: Full
- WDS Catalog: 00-05 Hour Section
- WDS Catalog: 06-11 Hour Section
- WDS Catalog: 12-17 Hour Section
- WDS Catalog: 18-23 Hour Section
- WDS Catalog With Precise Last Only
- WDS Catalog As An SQL Database (Original Code, Danley Hsu; Improved Code, Damien Mattei)
- WDS With Constellation And Bayer/Flamsteed Designation (When Applicable) Appended
- Format Of The Current WDS
- Notes File For The WDS
- References And Discoverer Codes
-
WDS Supplemental Catalog
- WDS Supplemental Catalog: Explanatory file
- WDS Supplemental Catalog: Summary
- WDS Supplemental Catalog: 00-05 Hour Section (All Data)
- WDS Supplemental Catalog: 06-11 Hour Section (All Data)
- WDS Supplemental Catalog: 12-17 Hour Section (All Data)
- WDS Supplemental Catalog: 18-23 Hour Section (All Data)
- WDS Supplemental Catalog: Format Of Files
- IAU Commission G1
-
Sixth Catalog Of Orbits Of Visual Binary Stars
- Full Page
- Introduction
- Orbit Grading Method
- Description Of The Catalog
- Catalog statistics
- Acknowledgments And References
- Orbital Elements: Html
- Orbital Elements: Text
- Orbital Elements: SQL
- Ephemerides:Html
- Ephemerides:Text
- Notes:Html
- Notes:Text
- References:Html
- References:Text
- Orbital Elements: Frame Version
- Formats Of Elements And Ephemerides Files
- Calibration Candidates
- Top 25 Orbit Calculators
- Master File Database
- Catalog Of Rectilinear Elements
- Fourth Catalog Of Interferometric Measurements Of Binary Stars
- The Delta-M Catalog
- IERS ICRS Center
- IVS (VLBI) Analysis Center
- IVS (VLBI) Analysis Center for Source Structure
-
Data Products
- Overview
-
IAU Double star center
- Overview
-
WDS Catalog
- WDS Catalog: Full
- WDS Catalog: 00-05 Hour Section
- WDS Catalog: 06-11 Hour Section
- WDS Catalog: 12-17 Hour Section
- WDS Catalog: 18-23 Hour Section
- WDS Catalog With Precise Last Only
- WDS Catalog As An SQL Database (Original Code, Danley Hsu; Improved Code, Damien Mattei)
- WDS With Constellation And Bayer/Flamsteed Designation (When Applicable) Appended
- Format Of The Current WDS
- Notes File For The WDS
- References And Discoverer Codes
-
WDS Supplemental Catalog
- WDS Supplemental Catalog: Explanatory file
- WDS Supplemental Catalog: Summary
- WDS Supplemental Catalog: 00-05 Hour Section (All Data)
- WDS Supplemental Catalog: 06-11 Hour Section (All Data)
- WDS Supplemental Catalog: 12-17 Hour Section (All Data)
- WDS Supplemental Catalog: 18-23 Hour Section (All Data)
- WDS Supplemental Catalog: Format Of Files
-
Sixth Catalog Of Orbits Of Visual Binary Stars
- Full Page
- Introduction
- Orbit Grading Method
- Description Of The Catalog
- Catalog statistics
- Acknowledgments And References
- Orbital Elements: Html
- Orbital Elements: Text
- Orbital Elements: SQL
- Ephemerides:Html
- Ephemerides:Text
- Notes:Html
- Notes:Text
- References:Html
- References:Text
- Orbital Elements: Frame Version
- Formats Of Elements And Ephemerides Files
- Calibration Candidates
- Top 25 Orbit Calculators
- Master File Database
- Catalog Of Rectilinear Elements
- Fourth Catalog Of Interferometric Measurements Of Binary Stars
- The Delta-M Catalog
- FRIDA
- 24 Hour Sessions
- UT1-UTC
- Global Solutions
FRAMEx Conceptual Background
Despite the extraordinary feat of producing the ICRF with decades of careful radio observations, issues remain. One notable issue is the relative lack of radio antennae at southern latitudes, leading to a deficit of southern-sky ICRF sources and overall worse astrometric precision at the corresponding declinations (see IERS ICRS background page, Figure 4). Another issue, which is the focus of the Fundamental Reference AGN Monitoring Experiment (FRAMEx), is the presence of statistically significant offsets between the radio positions of ICRF objects and their positions at other wavelengths, namely visual wavelengths where Gaia operates (Figure 1). While Gaia has its own internally-consistent reference frame (Gaia-CRF), this reference frame must be oriented into the ICRS. Intrinsic optical-radio positional offsets fundamentally limit the precision of this alignment, and even more so the positional accuracy of any particular ICRF source position across the electromagnetic spectrum.
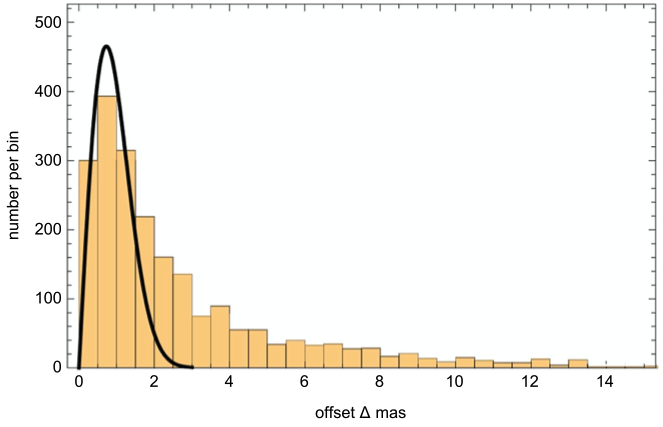
A natural explanation for optical-radio offsets is that the radio positions of AGNs, especially at the relatively low frequency at which the ICRF is defined (X-band, or 8.4 GHz), are dominated by lobes and knots in their extended jets. These often exhibit apparent faster-than-light (superluminal) motion, a peculiarity of special relativity when an object is moving close to the speed of light at certain angles with respect to the line-of-sight. When combined with differential brightening of knots in the jet due to its internal dynamics, this can lead to apparent changes in position over the span of only a few years. Moreover, the radio core of the AGN, which is most closely aligned with its true position, is often relatively faint compared to the more distant knots of emission due to synchrotron self-absorption, leading to the phenomena of frequency-dependent core shift.
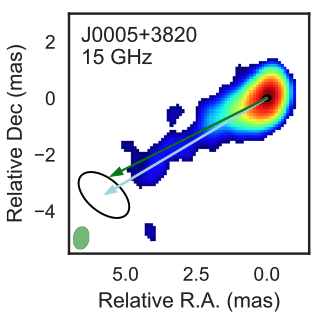
Not all radio-luminous AGNs exhibit large frequency-dependent positional offsets, but which particular AGNs do exhibit these offsets is time-dependent. For many objects with significant optical-radio offsets, jet activity does appear to be the explanation (Figure 2).
Additional factors that may contribute to frequency or wavelength-dependent positional offsets are AGN obscuration, which increases the relative contribution of the (possibly asymmetric) host galaxy to its apparent position at bluer wavelengths (i.e., at the 623 nm pivot wavelength of Gaia G), AGN intrinsic luminosity variability, which changes the relative contribution of the host galaxy at the same wavelength, the presence of dual or binary AGNs, which introduces random jitter in the position of their photo-center, and supernovae, which may temporarily affect the apparent positions of a handful of ICRF objects at visual wavelengths. Luminous AGNs are often found in “train wreck” systems (Figure 3), making determination of their true position difficult. All of these factors are notably time-dependent. As previously mentioned, radio core shift and jet superluminal motion can incur positional changes in the span of a few years.
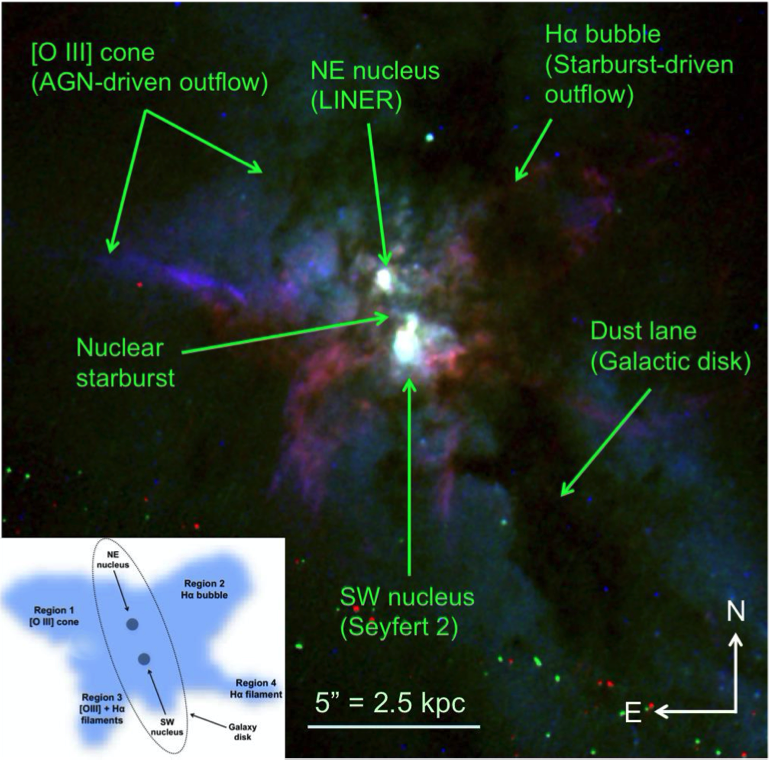
The same is true of changes in AGN obscuration (i.e., “changing-look” AGNs that transition between type 1 and type 2). AGN luminosity variability can occur in the span of hours, and AGNs can slowly ramp up or down in average luminosity over years and decades. This variability is often modeled as a damped random walk, but is fundamentally unpredictable. The time-dependence and fundamental unpredictability of AGN apparent position motivates dedicated multi-wavelength monitoring, which is a major component of FRAMEx. For example, the U.S. Naval Observatory (USNO) leverages its 50% timeshare of the VLBA to regularly observe the ICRF objects not only for its responsibility to monitor UT1-UTC, but also to monitor for morphological and positional changes. USNO also performs photometric regular monitoring of ICRF objects using the Deep South Telescope, a USNO asset at the Cerro Tololo Inter-American Observatory in Chile. Finally, USNO uses time-domain sky survey data from public facilities such as Pan-STARRS and the Wide-field Infrared Survey Explorer to characterize the variability of ICRF objects and correlate their properties with VLBI-Gaia positional offsets.
While the ICRF objects and their positional offsets are the central motivator for FRAMEx work, studies of nearby AGNs also make up a large portion of FRAMEx. The advantage to studying nearby AGNs is that their proximity allows for extremely high physical resolution studies. For example, the approximately milli-arcsec (mas; about 0.28 millionths of a degree) angular resolution of the VLBA in X-band subtends 8 parsec (about 26 light-years) for a typical ICRF object at a redshift of 1 (a comoving radial distance of about 3,300 Mpc, or 11 billion light-years), well outside the inner accretion region of AGNs (about 1 parsec). For a nearby AGN at 40 Mpc, the VLBA resolves a region of only 0.2 parsec, directly probing the accretion region and allowing for sensitive, high resolution studies of AGN physics. A complete sample of AGNs in the local volume out to 40 Mpc was selected from the Neil Gehrels Swift Observatory Burst Alert Telescope (Swift BAT) catalog, which is a full-sky catalog of hard X-ray AGNs with a uniform sensitivity limit, making the selection of a volume-complete sample straightforward. These objects have been the focus of regular monitoring with both the VLBA and the Swift X-ray Telescope (Swift XRT), in order to disentangle the relationship between accretion power in AGNs and the production of radio emission.
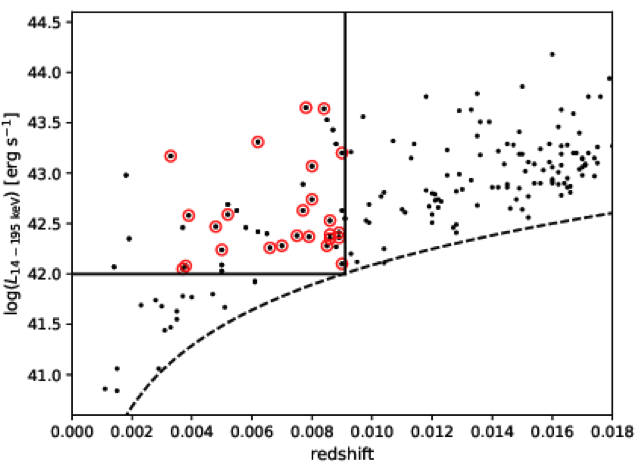
For more information on FRAMEx, see Dorland et al. (2020).
References
- Charlot, P., Jacobs, C.S., Gordon, D., et al. 2020, A&A, 644, A159
- Dorland, B., Secrest, N., Johnson, M., et al. 2020, “Astrometry, Earth Rotation, and Reference Systems in the GAIA era”, 165
- Fischer, T.C., Secrest, N.J., Johnson, M.C., et al. 2021, ApJ, 906, 88
- Kovalev, Y.Y., Petrov, L., & Plavin, A.V. 2017, A&A, 598, L1
- Makarov, V.V., Frouard, J., Berghea, C.T., et al. 2017, ApJL, 835, L30
- Müller-Sánchez, F., Nevin, R., Comerford, J.M., et al. 2018, Nature, 556, 345
- Türler, M., Paltani, S., Courvoisier, T.J.-L., et al. 1999, A&AS, 134, 89
3450 Massachusetts Ave NW,
Washington, DC 20392
Thursdays from 3-5pm Eastern Time, during
which time the website may be unavailable.